Interacting binary stars
- Dirk Goës
- Apr 2
- 7 min read
Stars that pull each other apart, merge, and explode
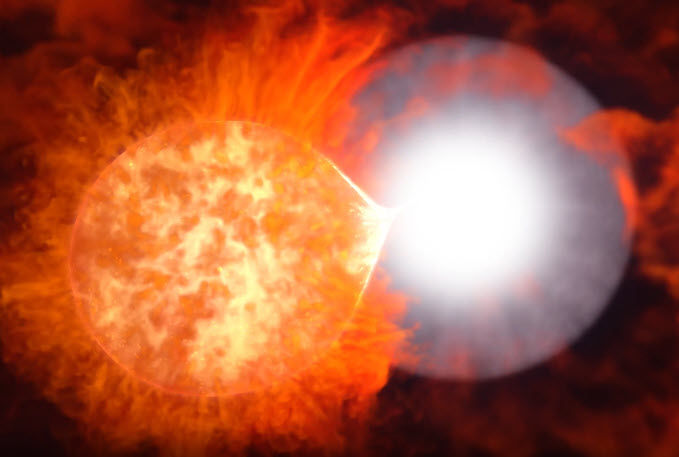
By Dirk Goës
A binary star system is one where two stars are gravitationally bound together and orbit each other in either circular or elliptical orbits. At least 50% of stars visible in the night sky and at least 50% of all stars are part of a multiple star system consisting of two or more stars orbiting each other. Binary stars, also known as double stars, are a favourite viewing target for many amateur astronomers. If you are interested in observing double stars through a telescope a good place to start is here.
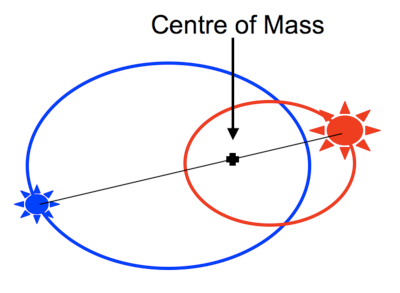
Some binary systems are simply two stars orbiting each other quietly. In many cases, however, the two stars interact. The most common example of this is by transferring mass from one star to the other. This transfer of mass can, for example, result in periodic thermonuclear explosions or in a supernova explosion which destroys one or both stars. These types of systems are called interacting binaries and occur when the stars are orbiting each other closely and when one or both stars are in an advanced state of evolution.
The transfer of mass can occur when one of the stars in the binary system expands to overfill its gravitational domain. The gravitational domain of a star is called its Roche-Lobe. Any gas outside the Roche-Lobe of the star may flow to its companion under the right conditions.
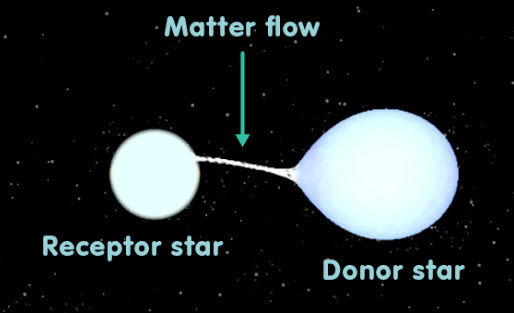
This article describes four examples of binary systems. One is the brightest star system in the sky, a non-interacting binary. The other three are interacting binary systems including a recurrent nova, an X-ray binary and a supernova.
Sirius

Sirius is the brightest star in the night sky and together with its companion Sirius B is an example of a non-interacting binary system. The two stars orbit each other over a period of about 50 years and on average are separated by 20 times the distance between the Earth and the Sun. The two stars quietly orbit each other without exchanging any material.
Sirius is a Sun-like star that is about twice as big as the Sun. Sirius B is a white dwarf star with a similar radius to the Earth but the mass of the Sun. A white dwarf is the left-over remanent of a star that has exhausted its supply of nuclear fuel and shed its outer layers. It is the final stage in the evolution of a Sun-like star.
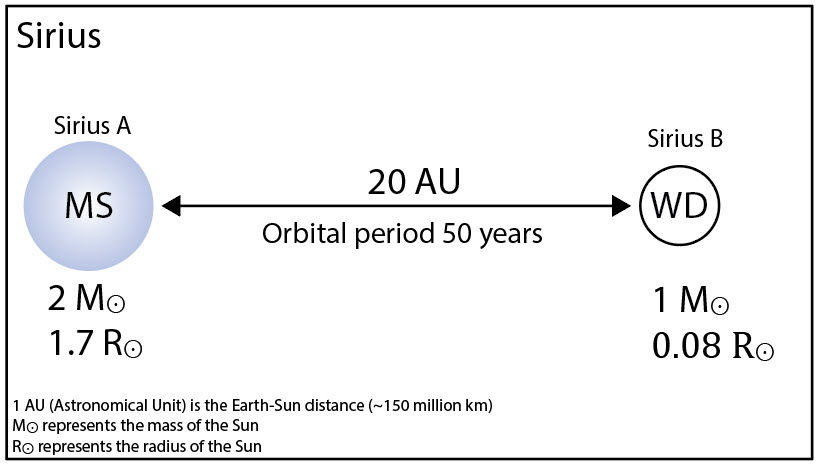
T Coronae Borealis
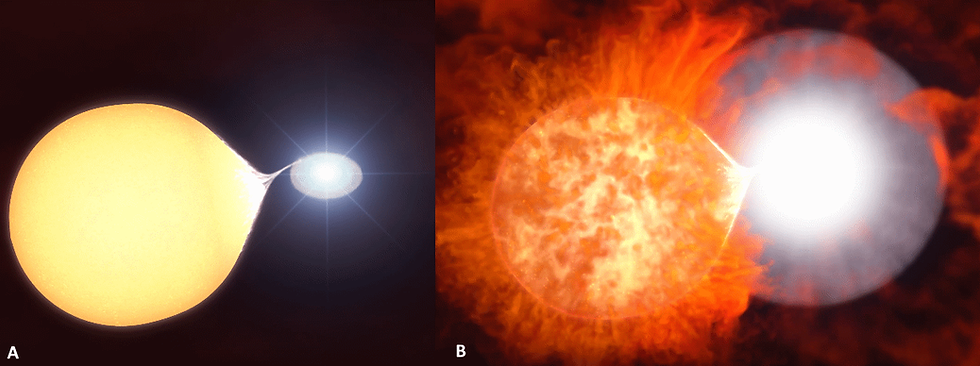
T Coronae Borealis (T CrB), also known as the Blaze Star, is an interacting binary system where the mass transfer from the red giant star to the white dwarf star results in a thermonuclear explosion on the surface of the white dwarf approximately every 80 years. The most recent explosion was observed in 1946.
Based on an observed pre-eruption dip in brightness the next explosion was predicted to occur in 2024 but did not take place. It is now predicted to possibly occur in 2025 or as late as 2027. In its quiescent state T CrB is not visible to the naked eye however when the explosion occurs it will be approximately as bright as Alphecca the brightest star in the constellation of Corona Borealis as shown in figure 6.
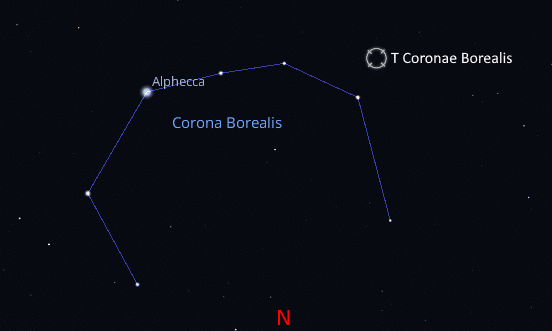
T CrB is a type of interacting binary system also known as a cataclysmic variable or a recurrent nova. They are characterised by the rapid accretion of hydrogen rich gas onto the surface of a white dwarf pulled in from the binary companion which is either a main sequence star or a red giant. Once a critical amount of material has been accumulated on the surface of a white dwarf a thermonuclear explosion occurs. This results in a tremendous increase in brightness that will slowly fade away over several months. The accumulation of material will continue, and the cycle will repeat.
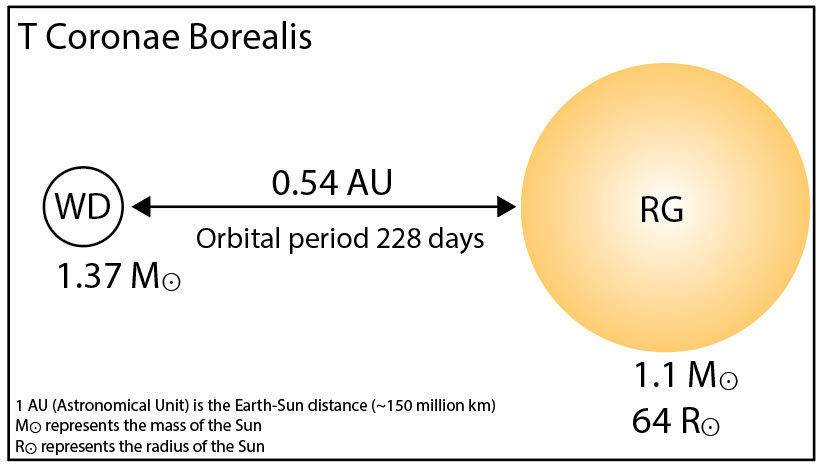
Cygnus X-1

Cygnus X-1 is an example of a type of interacting binary system called an X-ray binary. It consists of a black hole sucking material off its companion star, a blue supergiant. The material drawn from the blue supergiant is heated to millions of degrees as it forms an accretion disk around the blackhole and emits X-rays in the process.
Cygnus X-1 was the first blackhole ever detected and was co-discovered by Australian astronomer Louise Webster in 1972. While the blackhole itself is not visible its presence was determined and calculated from the X-ray emissions.
The blue supergiant star and the blackhole orbit each other every 5.6 days and the distance between them is less than the distance between the Sun and Mercury. The blackhole is 21 times the mass of the Sun. The blue super giant is 41 times the mass of the Sun and 22 times the size of the Sun.
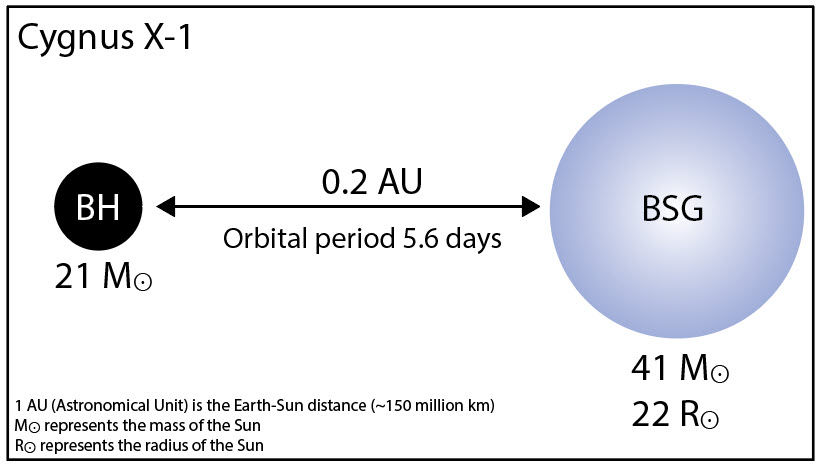
Supernova 2014J

In 2014 astronomers observed a supernova explosion in Galaxy M82 and named it SN 2014J. At approximately 11.5 million light years away, it was the closest supernova of its type recorded since 1972. Specifically, it was a Type Ia Supernova which involves the explosion of a white dwarf in an interacting binary system. Observations of Type Ia Supernovae were famously used to determine that the universe is expanding at an accelerating rate.
A Type Ia Supernova is different to a core collapse supernova which is caused by a massive star exploding and caving in to become a neutron star or black hole. The most recent nearby core collapse supernova was observed in 1987 and named SN 1987A. It occurred in the Large Mallengenic Cloud at approximately 168,000 light years away.
A Type Ia Supernova is thought to occur from a couple of different binary star configurations. One is like T Coronae Borealis, described above, where a white dwarf is pulling material from a red giant. However, in this case the material being accumulated on the surface of the white dwarf causes its mass to exceed the Chandrasekar Limit of 1.4 times the mass of the Sun. This results in a thermonuclear explosion and the complete destruction of the white dwarf.
The second possible configuration is when the binary system consists of two white dwarfs orbiting each other. In this scenario the two white dwarfs may spiral into each other causing them to merge. The merger results in a Type Ia Supernova explosion.
A study by Margutti et al. (2014) found that a lack of X-Ray emissions from this explosion indicate that the second scenario, a white dwarf merger, is a more likely cause of SN 2014J. This is because in the first scenario the shock wave from the explosion would be expected to heat up the surrounding material (pulled from the companion star) to a temperature that produces X-Rays.
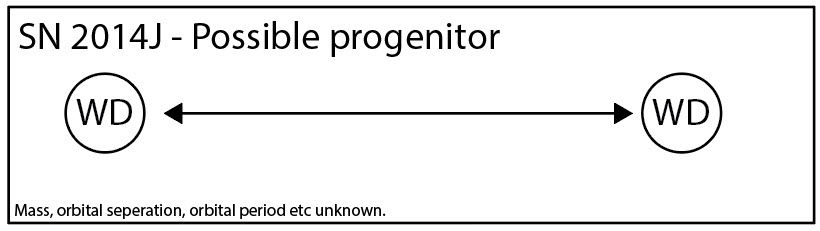
Many more binaries
This article has described a small selection of binary and interacting binary star systems. However, there are many more and many variations on those described above. For example, you may like to investigate black hole binaries or black hole-neutron star binaries and how the merger of these objects generate gravitational waves.
If you are interested in observing binary systems, you may like to train your telescope onto T Corona Borealis and see if you can one of the first to spot the nova outburst. Or you could attempt to observe U Geminorum, an interacting binary that has an outburst approximately every 105 days. Or you could attempt to spot Sirius B in the glare of Sirius.
留言